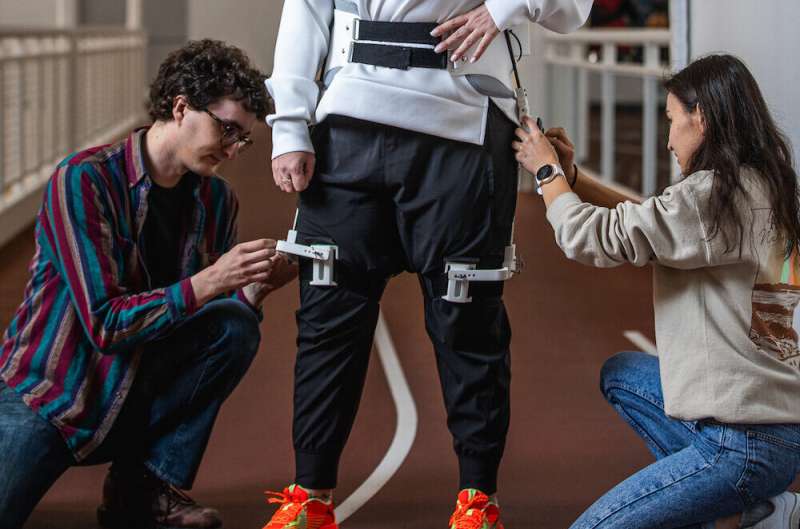
Meghan Huber wearing the hip exoskeleton with Mark Price and Banu Abdikadirova (credit: Derrick Zellmann). Credit: Derrick Zellmann
More than 80% of stroke survivors experience walking difficulty, significantly impacting their daily lives, independence, and overall quality of life. Now, new research from the University of Massachusetts Amherst pushes forward the bounds of stroke recovery with a unique robotic hip exoskeleton designed as a training tool to improve walking function.
This invites the possibility of new therapies that are more accessible and easier to translate from practice to daily life compared to current rehabilitation methods.
Following a stroke, people often experience walking asymmetry, where one step is shorter than the other. The study, published in IEEE Transactions on Neural Systems and Rehabilitation Engineering, reveals that the robotic hip exoskeleton has the potential to effectively train individuals to modify their walking asymmetry, presenting a promising avenue for stroke rehabilitation.
The approach employed by the robotic exoskeleton is inspired by split-belt treadmills, which are specialized machines with two side-by-side belts moving at different speeds. Prior research has shown that repeated training on a split-belt treadmill can reduce walking asymmetry in stroke patients.
Wouter Hoogkamer, assistant professor of kinesiology and author of the paper, has spent the last decade studying split-belt treadmills. “Split-belt treadmill training is designed to exaggerate a stroke patient’s walking asymmetry by running the belts under each foot at different speeds. Over time, the nervous system adapts, such that when the belts are set to the same speed, they walk more symmetrically.”
Unfortunately, there are limits to the benefits gained from treadmill-based training methods. “What is learned on a treadmill does not completely transfer to overground contexts,” says Banu Abdikadirova, mechanical and industrial engineering doctoral candidate and lead study author. “This is because walking on a treadmill is not exactly the same as walking overground.”
“The ultimate goal of gait rehabilitation is not to improve walking on a treadmill—it is to improve locomotor function overground,” says Meghan Huber, assistant professor of mechanical and industrial engineering and senior author on the paper. “With this in mind, our focus is to develop methods of gait rehabilitation that translate to functional improvements in real-world contexts.”
This proof-of-concept study showed that applying resistive forces about one hip joint and assistive forces about the other with their exoskeleton mimicked the effects of split-belt treadmill training in neurologically intact individuals.
Now that the research team has proven that the exoskeleton can alter gait asymmetry, they are eager to move their research into overground contexts that are more akin to the real world.
“Because our exoskeleton is portable, it can be used during overground walking,” says Mark Price, a postdoctoral researcher in mechanical and industrial engineering and kinesiology and author on the paper. “We can build upon the successes of split-belt treadmill training with this device to enhance the accessibility of gait training and enhance the transfer of training benefits into everyday walking contexts.”
The researchers also plan to expand their work by measuring the neural changes caused by walking with the exoskeleton and testing this new method on stroke survivors.
“A portable exoskeleton offers numerous clinical benefits,” says Abdikadirova. “Such a device can be seamlessly integrated into the daily lives of chronic stroke survivors, offering an accessible way to increase training time, which is critical for improving walking. It can also be used during early intervention in hospitals for improved functional outcomes.”
The robotic hip exoskeleton is just one of the innovative devices designed to study and enhance gait function developed by the collaborative team of undergraduate students, graduate students, and postdoctoral researchers from the Human Robot Systems Lab, led by Huber, and the Integrative Locomotion Lab, led by Hoogkamer.
“It is inspiring to witness the innovations that emerge when individuals from diverse backgrounds unite under a shared mission,” says Huber. “Only through this type of cross-disciplinary research can we engineer technologies that can have a meaningful impact on people’s lives.”